Rock Springs Uplift and Adjacent Areas (cont.)
2 Quaternary Volcanic Forms: Leucite Hills
2.1 Overview
Probably because of its flat-slab character, the subduction of the Farallon plate underneath the Wyoming craton was not accompanied by magmatism: in subduction dynamics, magmatism develops at a 110 km depth (the Benioff-Wadati zone). That's why there are no volcanic rocks allowing to date with precision the Sevier and Laramide orogenies. However, during Quaternary time, 14 volcanoes erupted during a pretty short period of time (from 3 Ma to 0.89 Ma, mostly between 0.94 and 0.89 Ma [40Ar/39Ar dating by Lange et al., 2000]), in a limited area on the eastern edge of the Green River basin and in the northern Rock Springs uplift. All these volcanic rocks are ultrapotassic lamproites and contain the uncommon leucite mineral (KAlSi2O6, a tectosilicate), hence the name Leucite Hills given to this volcanic field. Because of their young age and the aridity of the climate, erosion has not been very active and most forms have been preserved, except for a few necks deriving from the erosion of volcanic cones (e.g. Boars Tusk). Many volcanoes are tabular (mesas, e.g. North and South Table Mountains, Black Rock). The largest mesa is Zirkel Mesa, northeast of Superior: it is also the most recent and consists of several cones, separated by lava flows.
Click on image for full-size zoomable image
Figure 2-1. Map of the Leucite Hills volcanic features, Wyoming.
Red dots locate volcanic forms (volcanic cones, lava flows, plugs, dykes). Iddings Butte, Weed Butte and Hallock Butte are shown with one single dot. Some spellings vary from one source to another. Some names have changed: Spring Butte was first called Orenda Mesa (hence the rock name orendite), Badgers Teeth Twin Rocks, Deer Butte Cross Mesa (from the geologist), Cabin Butte Osborn Mesa, etc. Base map: Rock Springs 1:250,000 topographic map (USGS).
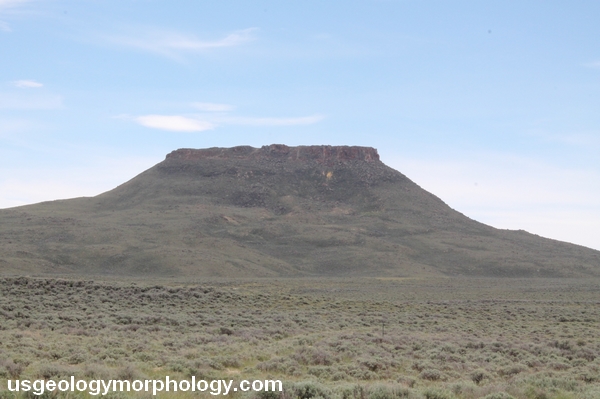
Figure 2-2. South Table Mountain, Wyoming
A volcanic mesa. The flat top is made by several lava flows.
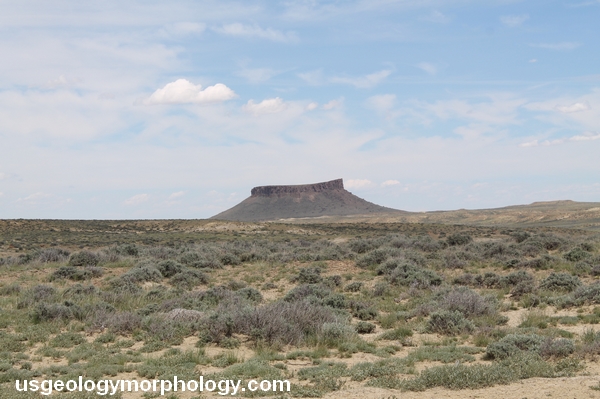
Figure 2-3. Black Rock, Wyoming.
Another mesa. View to the east. A volcanic plug for Cross and Schultz (1912), it has been interpreted as a basal pyroclastic unit overlain by a lava flow by Ogden (1979).
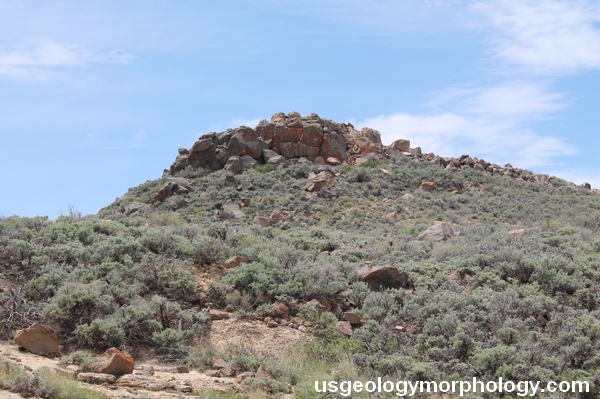
Figure 2-4. Southeastern edge of Zirkel Mesa.
Edge of an orendite lava flow on southeastern Zirkel Mesa, Wyoming. Erosion has divided lava into blocks which slide down the talus.
2.2 Petrogenesis
As early as the end of the 19th century (Kemp, 1897, Kemp and Knight, 1903), the ultrapotassic volcanic rocks of the Leucite Hills were analyzed and named wyomingite, orendite and madupite. Since then, two more rock types have been added and they have been renamed according to the IUGS classification as follows:
-diopside-leucite-phlogopite lamproite (wyomingite);
-diopside-sanidine-phlogopite lamproite (orendite);
-olivine-bearing diopside-sanidine-phlogopite lamproite (olivine orendite);
-diopside madupitic lamproite (madupite);
-transitional madupitic lamproite.
The two commonest rocks are wyomingite and orendite; phlogopite forms phenocrysts, while the groundmass is made of leucite, diopside, sanidine, and glass. Both have the same chemical composition, but a different mineralogy, due to different conditions of crystallization; as can be seen in the list above, wyomingite has leucite and little sanidine, while orendite has sanidine and little or no leucite. Madupite is quite different with diopside phenocrysts enclosed by poikilitic crystals of phlogopite, the whole set in a groundmass of diopside, apatite and leucite (Carmichael, 1967).
They are all lamproites, pretty rare rocks, which are ultrapotassic K2O / Na2O > 2 and peralkaline (K2O + Na2O) > Al2O3. Unusually, they are both enriched and depleted. They have a high Mg#, high concentrations of compatible elements such as Ni and Cr, in LREE (light rare earth elements) and incompatible elements (K, Ba, K, Ti, Rb, Sr, F, Zr). On the other hand, they are depleted in Al, Ca and Na.
Three questions need then to be resolved: (1) the petrogenesis of such unusual rocks, (2) the origin of magma, (3) the cause of the volcanic event.
After the early mineralogical and chemical descriptions of the Leucite Hills rocks by Zirkel (1876), Kemp (1897), Kemp and Knight (1903) and Cross and Schultz (1912), more modern and comprehensive studies were published by Smithson (1959), Johnston (1959), Yagi and Matsumoto (1966), Carmichael (1967) and Ogden (1979), but none of them could reach a definitive theory of the origin of these rocks.
Powell and Bell (1970) confirmed that the mantle was the source of the lavas, after their 87Sr/86Sr ratio, ranging from 0.7055 to 0.7070. Several melting experiments were conducted by Sobolev et al. (1975), Barton and Hamilton (1978, 1979) on the genesis of madupite, wyomingite and orendite.
In 1981, Kuehner et al. showed that the Leucite Hills lamproites petrogenesis could be modeled on the systems KAlSiO4 (kalsilite) - Mg2SiO4 (forsterite) -SiO2 - CO2 and KAlSiO4 - MgO - SiO2 - CO2 - H2O. They would have derived from the same K-enriched peridotite. In dry conditions madupite would have formed at 24-27 kbar (84-94 km) and wyomingite-orendite at 14.5-15.5 kbar (51-54 km). Under conditions of CO2-saturation with the presence of H2O, madupite would have been generated at 24 kbar i.e. 84 km, wyomingite and orendite at 14-17 kbar i.e. 49-60 km. In 1982, Barton and Hamilton perfected their previous experiments and established that under water-undersaturated conditions, a phlogopite-garnet-lherzolite or a peridotite enriched in K and incompatible elements could produce an orendite melt where, as it ascended from a 27-30 kbar depth (~100 km) and cooled, phlogopite, diopside, leucite and sanidine would crystallize. Sharygin and Bazarova (1991), in a similar study on wyomingite, reached similar conclusions on the crystallization sequence. Moreover, Gunter et al. (1990) and Petrushin et al. (2004) explained the common layering of wyomingite and orendite in the same volcano by the role of H2O, sanidine being more stable than leucite under high H2O activity.
Mirnejad and Bell (2006) proposed a three-step origin for the magma which the Leucite Hills lamproites originated from. Archean magmatism first depleted the SCLM (sub-continental lithospheric mantle) in Al, Ca and Na, leaving a refractory harzburgite. Ancient metasomatism, at least 1 Ga old, probably even older (late Archean to middle Proterozoic), related to subduction or continent collision, enriched the mantle in LREE, Nd/Sm and Rb/Sr ratios. The last two would explain the current strongly negative εNd (previously measured by Vollmer et al., 1984) and the moderate 87Sr/86Sr ratio, which both place the madupite and the phlogopite lamproites (wyomingite and orendite) in the enriched quadrant of the 87Sr/86Sr-εNd diagram.
Finally, for Mirnejad and Bell (2006), recent metasomatism, in the last 100 My, would have enriched the mantle in K. The absence of correlation between εNd and K2O abundance shows they relate to two distinct events. The younger metasomatic event is confirmed by the δ18O of the phlogopite phenocrysts, which proves some hydrous fluid activity, in the absence of crustal contamination. For some researchers the flat slab subduction of the Farallon plate at a relatively shallow depth could explain this metasomatic event, but the authors note that the subduction zone should have extended 1200 km from the continental margin. Other workers proposed the role of the Yellowstone hotspot, but it is 300 km to the north at began its activity only 19 My ago much further to the west. Instead Mirnejad and Bell suggest the effects of back-arc extension and lithospheric thinning related to the Farallon plate subduction.
Lange et al. (2000) hypothesized that the elevation of mantle temperature south of the Cheyenne belt under the Colorado plateau (Henstock et al., 1998) could have propagated under the southwest Wyoming craton. On Obrebski et al.'s (2011) tomographic maps at a 25 km depth, the Colorado plateau seismic low-velocities extend northward to the Rock Springs uplift and the Leucite Hills area; at a 50 km depth, the same areas display average-to-low velocities. Levander et al. (2011) proposed a model for the Colorado plateau where the mantle was removed by downwelling, delaminating the lower lithosphere, both being replaced by hotter asthenosphere. From these two studies, Ishikawa and Kawai (2015) suggested that transient upwelling asthenosphere under the neighboring southwest Wyoming craton could have caused the Leucite Hills magmatism.